Nuclear fusion holds the promise of an inexhaustible supply of energy for our economy. Tremendous effort is under way around the world to make nuclear fusion a technological reality. But will it ever become economically viable? Can nuclear fusion be commercialized into a competitive power source? When I first learned about nuclear fusion decades ago, the joke was "nuclear fusion is thirty years away from commercialization—and always will be." Nuclear physicists have made tremendous progress in the last decades, and nuclear fusion may well become a technological reality. The cutaway diagram below shows ITER, the international cooperation projection located in France that is supported by the European Union, the United States, China, Japan, Korea, India and Russia. ITER is designed to produce 500 Megawatt (MW) of power when it becomes operational after 2025, producing a ten-fold return on its energy input. (However, this power output ratio has been disputed; it appears to be overly optimistic.) Yet, even if ITER is successful, this will not prove that the technology is competitive economically in terms of levelized cost of electricity (LCOE). What will it take to get there? Will nuclear fusion ever reach grid parity? In the near future, nuclear fusion is not a solution for our climate crisis. It will offer too little too late to help. Solar power along with hydro and wind, as well as geothermal power, are the answers we need to reach the targets of the Paris Agreement.
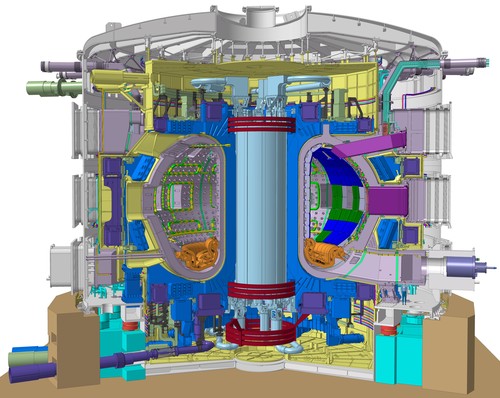
Nuclear fusion faces not one but two challenges. The first is to reach stable confinement of the fusion plasma at critical temperatures—about 100 million Kelvin—and pressures. The easiest way to achieve that is to "burn" a mix of deuterium (D) and tritium (T), two isotopes of hydrogen (H). There are superior fuel cycles, but they are more difficult to reach. Thus there is the second challenge: providing a way to generate a steady supply of tritium in the absence of natural sources. This second challenge is the Achilles Heel of nuclear fusion, because the proposed methods may not reach a critical threshold for self-sustaining supply. Without it, nuclear fusion has little chance of achieving grid parity.
‘Nuclear fusion's tritium dilemma will keep nuclear fusion very expensive for long.’
Most nuclear fusion designs employ a deuterium-tritium cycle, which requires the lowest temperature-pressure combination to ignite the reaction. The two hydrogen isotopes have rather different prices. Deuterium can be won by separating heavy water (D2O) from ordinary water (H2O) through various processes such as the Girdler sulfide process. Tritium (T) is a radioactive isotope with a 12.32 half-life and thus does not occur naturally. Any existing supply decays rapidly. The world supply of tritium is not sufficient to cover the needs of future power plants. The ITER reactor will buy the tritium from global inventory, but its planned pre-commercial successor DEMO will need 300 grams of tritium per day to produce 800 MW of power. Tritium is currently produced in nuclear fission reactors, for example in those that use heavy water (deuterium) as a moderator. For nuclear fusion systems, a different process is envisioned that is called tritium breeding. Lithium-bearing ceramics are bombarded with neutrons that in turn generate helium and tritium. The general idea is that tritium breeding generates a sufficient fresh supply of tritium for the power plant without the need for an external source. For this to work, a nuclear fusion plant needs to breed at least as much tritium as it consumes.
‘Commercialization of fusion energy depends on self-sustaining tritium breeding.’
The economics of nuclear fusion power plant hinges entirely on tritium breeding because without it, commercially available tritium remains one of the most expensive substances on Earth, at $30,000 per gram. Without effective tritium breeding, nuclear fusion has a tritium dilemma that will keep this power source expensive for a very long time. Consider the DEMO reactor of 800 MW power and 300g of tritium per day. At $30,000 per gram, this would cost $9mio per day or $468.75 per MWh, about nine to ten times the LCOE for current sources! The successful development of tritium breeding is an indispensable necessity for commercializing fusion energy.
Technologically, lithium blankets such as those planned by ITER remain in the conceptual design stage and have not progressed past the point of initial experiments. The key to figuring out the economics of the tritium dilemma is ascertaining that a tritium breed ratio (TBR, the amount of tritium red to tritium burnt) exceeds about 1.1, which provides a margin to allow for tritium decay during the time it takes to reprocess it. Theoretically, TBRs of about 2 are possible, but engineering issues will limit TBRs to much lower numbers. To achieve a high TBR, the natural mix of lithium has to be enriched in the 6Li isotope. To make one gram of tritium requires about 2 grams of 6Li, which is costly to make as well. Tritium prices are expected in the best case scenario to remain in the $10,000–$30,000/gram range (McMorrow 2011). Zhen and Todd (2015) project TBRs for ITER's planned successor DEMO that could become viable, but these remain design studies that have not been tested in ITER. LCOE projections based on self-sustaining tritium breeding (Entler 2018) put the proposed DEMO generator at about $160/MWh, significantly above the global average of $56 and $127 for onshore and offshore wind, or $85/MWh for solar photovoltaics and $72/MWh for geothermal (IRENA 2019). The cost of these renewable energy sources is expected to drop further.
In Canada, Burnaby-based General Fusion has received much attention lately as it represents a rather unique approach to nuclear fusion. Unlike the big international ITER project that uses a tokamak confinement of the plasma, General Fusion's design uses magnetized target fusion with steam-driven pistons to mechanically compress a vortex of liquid metal. It will employ the deuterium-tritium cycle. The company has claimed to be able to achieve a TBR of 1.6 with natural lithium, but this remains a bold claim until it can be ascertained empirically.
In principle, nuclear fusion can rely on alternative fusion cycles. The deuterium-deuterium (D-D) cycle generates a mix of tritium and helium-3 and ignites at a higher temperature than the deuterium-tritium (D-T) cycle. The neutronicity of the D-D process is slightly better, but not much. Too many neutrons damage the containment vessel, and reduce its useful lifetime. Processes with very low neutronicity, known as aneutronic fusion such as the proton-Boron or the deuterium-helium-3 process, either ignite at temperatures (over 1 billion Kelvin) that will remain out of reach for many decades yet or require helium-3 that is rather scarce on Earth (it is abundant on the Moon, however). This has not stopped a small company TAE Technologies in California from trying to design a prototype that uses the proton-boron cycle, which only produces three helium nuclei. Not much is known about the company's progress, and it has not reached break-even fusion yet.
What does all of the above imply for public policy? In terms of devoting precious research dollars, nuclear fusion is a long shot. There is no realistic prospect that nuclear fusion will become commercially viable any time before 2050. ITER and its national cousins, such as Germany's Wendelstein 7-X or Britain's recently-proposed STEP, will require massive public funding for decades to come. A case can be made for such long-term research projects, but it remains basic rather than applied research. The huge capital needs for the ITER project stretch even the budget constraints of the United States Department of Energy. To deal with our worsening climate crisis, investments are needed into renewable energy and into a smarter electricity grid. There is no shortage of investment needs, and unlike nuclear fusion, they have an attractive return on investment.
Further readings and information sources:
- Henry Fountain: A Dream of Clean Energy at a Very High Price, The New York Times, 27 March 2017.
- Slavomir Entler, Jan Horacek, Tomas Dlouhy, Vaclav Dostal: Approximation of the economy of fusion energy, Energy 152, 1 June 2018, pp. 489-497.
- S. Zhen and T.N. Todd: Study of Impacts on Tritium Breeding Ratio of a Fusion DEMO Reactor, UK Atomic Energy Authority, 2015. Subsequently published here.
- Matt McGrath: Nuclear fusion is 'a question of when, not if', BBC, 6 November 2019.
- Fusion power is attracting private-sector interest, The Economist, 4 May 2019.
- Dino Grandoni: Start-Ups Take On Challenge of Nuclear Fusion, The New York Times, 25 October 2015.
![[print]](print.png)
![[Sauder School of Business]](logo-ubc-sauder-2016.png)
![[The University of British Columbia]](logo-ubc-2016.png)